I mentioned in a previous blog post that water inflow, retention and drainage can be manipulated in planting beds to reach particular functional goals. These manipulations can be executed in a variety of ways, on a variety of different sites, meaning that the construction and placement of rain gardens influences many other site factors besides water availability.
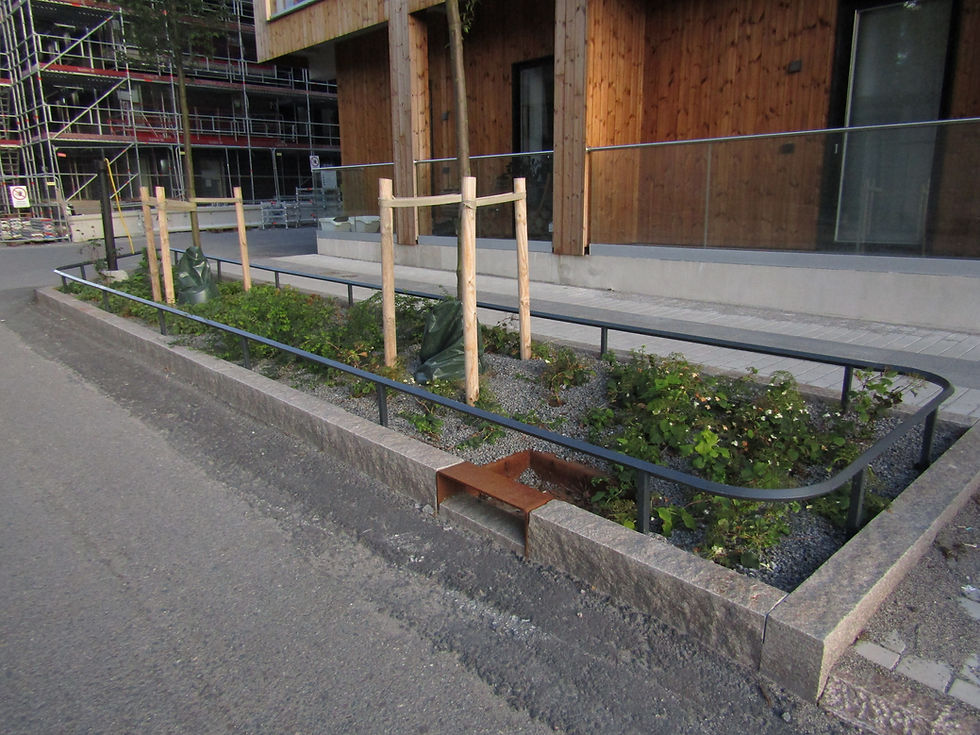
In this post we’ll look at how space-, water-, oxygen-, nutrient-, and light availability as well as microclimate factor in on plant life. I also hope to be able to demonstrate the interconnectedness of the factors above, as well as why you can't compensate for one resource with another indefinitely. Before we get into the specific technical nitty-gritty of how resource availability can be manipulated in rain gardens, I would like to first get into the heart of why we might want to do these manipulations on planting beds in the first place.
The engineering-minded reader might say "to better transport, retain, detain, infiltrate and treat water!" They are wrong. All of the functions above can be performed by solutions that include no plants or planting beds. Water transport can simply be a function of gravity, or maybe a pump in a pipe system; Water can be retained in any water-proof vessel; If the vessel has a hole in it, it will still have a detaining function; And water treatment may be done by adding chemicals to it or by filtering it through fabric or soil, just to name some options. No, the manipulations I will suggest are mostly for the benefit of plants. Because we are talking about plantings. You know. With living plants.
Before you boo me off the stage, hear me out. I am not advocating for separating vegetated areas from stormwater again, and neither am I saying that we must always and everywhere prioritize the health and well-being of urban vegetation. But what is sometimes forgotten or misunderstood - especially from the part of engineers - is that these combined solutions can be very stressful environments for plants, and that they will develop and perform worse under pressure. We have had this discussion before: stresses, and especially multiple simultaneous long-lasting stresses, eat at plants as they eat at people. That is why it is sometimes necessary for plant health to diminish the intensity or types of stress in average circumstances, so that they can contribute positively to the multifunctionality of a site and make it through extreme circumstances better.
To better understand why different stresses can be so detrimental to plants, let's look at plant anatomy and physiology. Just like other organisms, plants are made of cells. The main specialties of plant cells are their thick cell walls that could be considered to be "plant bones", and the chloroplasts that transform water and carbon dioxide into sugar and oxygen during photosynthesis. Other than that, they are largely similar to animal cells in their basic structure in the sense that they consist mostly of water, they "breathe" as a part of their energy cycles, and that they have different kinds of smaller organs that make hormones and building blocks for different parts of the plant. Just by looking at the cellular structure, we can understand the basic needs of a plant: Water is indispensable for overall cell function and photosynthesis; hormones and other specialized components are synthetized from nutrients; oxygen is involved in the cellular "breathing" process; Carbon is crucial a part of sugar molecules and plant tissues*; and light is required for creating sugar, which both powers the cells and is a component of cell walls, among other things.
If the cellular level feels a bit too abstract, it's okay for now. The important thing to understand at this phase is that all of the environmental factors that influence the availability of these essentials have consequences for the plant on a cellular level, which reflects back into plant performance that can be observed with the naked eye**. We'll concretize this further by looking at planting situations that differ from each other with regards to available space above- and underground, soil conditions, available light and microclimate.
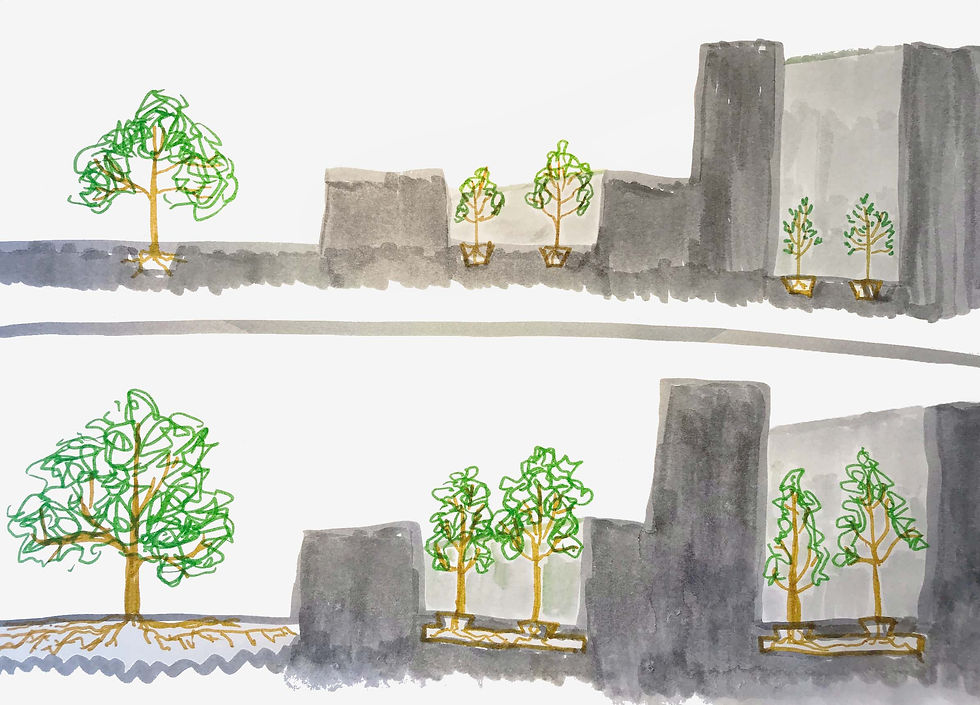
Space belowground is decisive for the plant's capability to forage for most of the essential resources. Root systems spread out to gather as much water and nutrients as possible, while keeping to areas where there is enough air in the soil to keep the cells oxygenated and alive. Root system configuration and spread is more flexible than canopy spread, and as a rule of thumb the root system of a tree has roughly thrice the diameter of the crown. On the other hand, the roots go where they can, and where they find the resources, which means that root systems in reality are very rarely symmetric or evenly distributed. Another function of the root system is to keep the plant tied to the ground, in some cases even in storm winds and landslides. The majority of plants’ root systems can be found at a depth of 30-60 cm belowground, after which resource availability of oxygen and nutrients often becomes very low. Water, on the other hand, may be found much deeper, and so some plants have adapted root systems that reach lower than most. One example of this would be the quaking aspen (https://en.wikipedia.org/wiki/Populus_tremula), whose roots often look for flowing groundwater. Many plants also adapt their root system depth to prevailing site conditions, although this doesn't mean that you can expect a white clover (https://en.wikipedia.org/wiki/Trifolium_repens) to reach all the way down to the bottom of a 1-meter deep tree pit. In other words, small planting bed area can’t generally be compensated for by increasing depth.
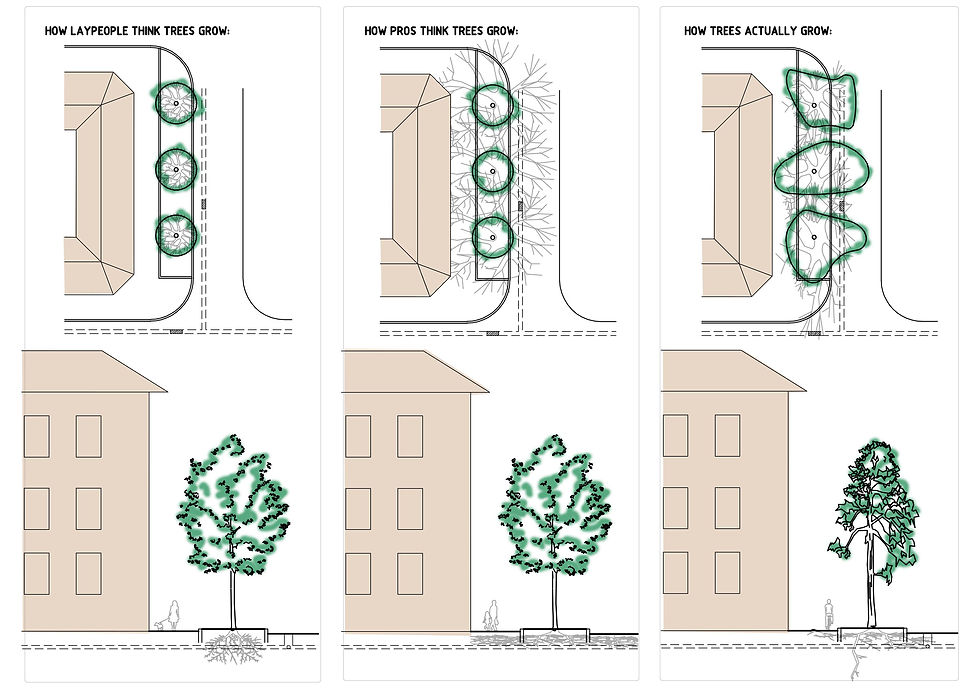
The first resource that most people think about when talking about plant needs is usually water. The water content of plant cells in different tissues varies, but generally speaking soft plant tissues can consist of up to 90% water. Basically, water keeps cells alive, whether it is a microbe, animal or plant cell. In plants water is also a main component for sugar created in photosynthesis. When a plant cell dries out, it implodes to death. For soft tissues this is a major problem, as water is as important for keeping the plant standing up as the cell walls are. In hard tissues this is not a problem, as their specialized cells keep their form even after drying out. This is the basic mechanism of tree and shrub growth: living cells on the outskirts of the branch or stem are continually dying to make the hard wood that makes up most of their volume.
Besides a simple lack of plant available water in soil, high concentrations of nutrients, salts and other watersoluble chemicals can contribute to a plant drying out. Water is taken up passively through a combination of intake at the roots and removal in leaves and shoots. This mechanism is also how nutrients are taken in and transported in the plant. The passive uptake via osmosis depends on the fact that soil water includes less nutrients and salts than the root tissues, otherwise the water leaks out of the plant into the soil instead. Similarly, soil drought and poor nutrient availability often go together, as there is not much water for nutrients to dissolve into. Wet soils can be either rich or poor in nutrients; in the latter cases you can often find carnivorous plants that eat insects to gather nutrients.
As mentioned before, nutrients are used to synthetize materials for different plant tissues. Nitrogen, phosphorus and potassium contribute most to different plant tissues, and are often called macronutrients to separate them from the 14 other essential elements that are needed in much smaller amounts. All of the three elements participate in a great number of tissues and metabolic compounds, so I'm only naming a few of their most obvious uses. Plant DNA and RNA (as all DNA and RNA) is largely made of nitrogen compounds. Nitrogen also makes a large contribution to the formation of chloroplasts. So nitrogen deficiencies hinder photosynthesis, cell division and molecule synthesis. Phosphorus participates in storing and relaying genetic information as well, as well as makes up a large part of the compounds that transport energy between different parts of the cell. Potassium is especially important in regulating water transportation and keeping the nutrient concentration in plant cells high enough for optimal operation. Nutrient deficiency leads to stunted growth, higher susceptibility to pests and diseases, lower numbers of flowers and fruit, and finally death.
Animals capture oxygen by breathing it into their lungs, where the oxygen is absorbed into the bloodstream and transported throughout the body. Plants don't have lungs, and most of them also lack oxygen transportation systems. Instead, they breathe with all of their (living) cells to capture (and release!) both oxygen and carbon. The photosynthetically active parts of plants -or the "green bits", in scientific terms - usually only need to breathe in the night, as during daytime they can utilize the oxygen that they produce as a side product of photosynthesis. Other plant tissues breathe around the clock. For the aboveground parts of plants this is generally not a problem***, but belowground the situation is more complicated. Soil contains air, although the amount can vary heavily based on soil type, degree of compaction and water content. Additionally, oxygen levels in soil decrease in the lower soil layers. To put it simply, more porous and drier soils closer to the ground contain the most oxygen. Flooded soils usually contain dismissible quantities of plant available oxygen, as do compacted soils. Flooding especially can make plants rot due to oxygen deprivation. So just like animals, plants can drown and suffocate, although it is more common that oxygen deprivation only kills of parts of the plants' root system than that the whole plant dies. Of course, even partial root system death can be detrimental to the plant as a diminished root system has lower water uptake potential, worse anchorage and a smaller area to gather nutrients from.
Space aboveground translates into growth potential for canopy volume****, which in turn can be used as a very rough proxy for light capture capability. How large the potential canopy volume is and the extent to which the volume can be realized is the sum of availability of aboveground space and essential resources, the plant's inherent resource requirements, and the capacity of the plant to utilize the available resources. Inherent resource requirements are usually species-specific: some plants tolerate lower amounts of light, for example, thanks to specific adaptations in their cellular metabolism. Alternately plant species have externally obvious ways to make the most of the available resources, for example by using large leaves to capture as much light as possible. The third way for a plant to make the best of the situation is to make individual adjustments, for example by focusing on increasing its height to be able to reach the sun, or by placing the main bulk of its crown in spots with more daylight hours. The fourth mechanism, timing of growth to coincide with the lightest period of the year, is especially typical for herbaceous spring ephemerals, like the yellow star-of-Bethlehem and wood anemones .
Lookit them spring ephemerals.
As mentioned before, chloroplasts use energy from sunlight to produce simple sugar molecules, glucose. Since plants do not eat, photosynthesis is the main way for them to produce glucose as food for the cells. In other words, light deprivation makes plants starve! And as we all know, starving - or even plain hungriness - is not conducive to growth. On a cellular level lack of glucose means at least two things. First, without glucose the cells become energy-deprived and can't produce hormones or materials for different plant tissues, which leads to decreased resources for producing flowers and fruit, for example. Second, glucose in itself is a raw material for plant tissues, such as cellulose, hemicellulose, and pectin, which combine with lignin to make up plant cell walls and keep the plants standing. No glucose, no new plant cells, no new growth. So from this point of view it is in the plants' interests to maximize their canopy size.
Microclimate, that is to say air temperature, air moisture, and wind conditions, influence water flow through the plant. Water is removed from the plant through respiration - just like animals, plants remove excess water and carbon dioxide from their cells as a part of their breath cycle. Another mechanism is evapotranspiration, where water flows through the whole plant, from root to leaf, and is passively transported back into the air. This mechanism keeps water flowing in a plant, and can to an extent be regulated by specialized plant cells. Ambient heat, heating from direct solar radiation, dry air, and wind high winds accelerate the process, as they increase evaporation from leaf surfaces. If water availability belowground is good, this hastened water circulation is mostly beneficial to the plant as it also means more effective uptake of nutrients with the water. If the soil is low on water though, this type of microclimate dries plants out fast. The effects of microclimate on plants should also serve as a good example on how everything is connected; The sum of conditions above- and belowground dictate site stresses and plant life.
Often in projects we take a plain stormwater management perspective to rain gardens, and so nutrient-less, highly porous, mostly dry and relatively slowly draining planting beds are considered ideal. Usually the required volume of planting substrate for storing adequate amounts of water is also smaller than the volume of soil required for adequate plant growth. Finally, stormwater management is light-independent, unlike plant growth.
Stormwater is transient, mobile, and above all not alive. Plants, on the contrary, cannot move elsewhere in search of better living conditions. Individual plant needs must always be satisfied hyperlocally, whereas stormwater management is ideally multiscalar. We also need to have plants where people are to benefit from local ecosystem services; there will be no "livable cities" or "urban nature-based solutions" without plant life!
If we want to keep combining urban vegetation with stormwater management we need to find project-specific compromises between the needs of plants and the ideal (and often cookie-cutter) solutions for transporting, retaining, detaining, infiltrating and treat water. In the upcoming posts I will give some ideas for resolving these conflicts.
* Carbon deprivation is very very rare for terrestrial plants, since air contains plenty of it. Because of this, I'm not going any deeper into this question, but keep in mind that the carbon capture potential of plants is directly tied to their capacity to synthetize carbon dioxide into plant tissues. The bigger plant and the more slowly degradable plant tissues, the larger is the long-term carbon capture potential.
** Or with the help of glasses. Or as can be observed via touch and smell. Different options.
*** Unless somebody had decided to cover them with airproof paint or plastic. Don't do that.
**** I'm using tree-centric language for the sake of simplicity. Obviously, herbaceous plants can photosynthetize with all of their green parts.
Comments